Chitosan is a natural multifunctional biopolymer obtained by partial deacetylation of chitin, with a complex double helix structure composed of 2-acetylglucosamine and 2-aminoglucosamine chitosan. Chitosan is widely present in crustaceans such as shrimp and crabs, algae and plants, and large fungi such as mushrooms. It has a wide range of sources and abundant resources, and is the second largest class of polymer compounds after cellulose. Chitosan has good antibacterial, biocompatibility, film-forming, and biodegradable properties. Films made from chitosan as a matrix exhibit excellent antibacterial, water vapor, and oxygen barrier properties.
Liu Mengqi, Lv Rui, Zhou Hui, and others from the School of Food Science and Engineering at Dalian Ocean University outlined the antibacterial mechanism of chitosan and the enhancement of its antibacterial activity. They particularly elaborated on the application of chitosan edible antibacterial packaging in the food industry, summarizing the research progress of chitosan as an antibacterial agent from multiple perspectives, including basic research and practical applications, in order to provide reference for the application of chitosan in food packaging.
01
Antibacterial mechanism of chitosan
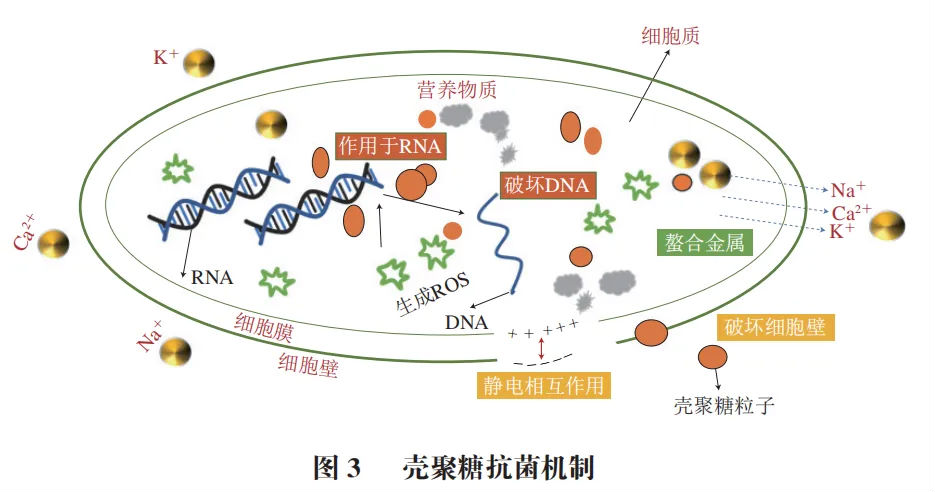
Chitosan has broad-spectrum antibacterial properties and significant inhibitory effects on various microorganisms such as bacteria and fungi, as shown in Figure 3. Its antibacterial mechanism mainly includes disrupting cell membrane permeability, affecting bacterial cell phospholipid and protein synthesis, chelating metal ions, etc.
1.1 Destruction of bacterial cell walls and alteration of cell membrane permeability
The cell wall and membrane of bacteria have the functions of protecting microorganisms from mechanical damage, maintaining osmotic pressure, and preserving cell morphology. The - NH3+in chitosan molecules carries a positive charge and can be adsorbed onto negatively charged bacteria through electrostatic interactions, thereby disrupting the integrity of the cell wall, increasing the permeability of the cell membrane, and causing osmotic imbalance, leading to the leakage of cell contents and a decrease in cell biological activity. The ultra long molecular chain of ultra-high molecular weight chitosan can encapsulate and bind to Escherichia coli and Staphylococcus aureus, causing cells to gradually rupture and decompose, greatly enhancing its antibacterial activity.
1.2 Effects on bacterial cell nucleic acid replication and protein synthesis
The synthesis of proteins and mRNA is the most fundamental life activity in bacterial cell growth. After entering the cell, chitosan can adsorb and bind with negatively charged proteins and nucleic acids, inhibiting the synthesis of proteins and mRNA, affecting the normal physiological functions of bacterial cells, and thus inhibiting bacterial growth and reproduction.
1.3 Chelated metal ions
The - NH2 and - OH in chitosan have certain metal adsorption capacity, while the outer membrane of Gram negative bacteria contains multi anionic lipopolysaccharides composed of divalent cations such as Mg2+and Ca2+. Therefore, chitosan can selectively bind to metal ions and essential nutrients in bacteria, thereby inhibiting the production of bacterial toxins and the growth and development of microorganisms.
02
The main ways to improve the antibacterial performance of chitosan
2.1 Chemical Modification
Chitosan is an alkaline polysaccharide with poor water solubility, which greatly limits its application. Through carboxymethylation, alkylation, sulfonation, and quaternization reactions, charged or hydrophilic groups can be introduced onto the surface of chitosan to modify - NH2 and - OH, improve its water solubility, and promote its antibacterial activity. Introducing carboxymethyl groups onto the - OH or - NH2 of chitosan can improve the surface hydrophilicity of chitosan molecules, as shown in Figure 4A. After carboxymethylation modification, the solubility of chitosan is improved, and the presence of carboxyl groups lowers the pH value of the reaction solution, resulting in more - NH2 protonation into - NH3+, which increases the number of antibacterial groups; At the same time, due to the formation of intramolecular or intermolecular hydrogen bonds between - COOH and - NH2 inside, the molecular chains can stretch out and come into full contact with bacteria, improving the antibacterial performance of chitosan. The antibacterial effect varies depending on the substitution position of carboxyl groups. This is because the - NH2 of chitosan is crucial for its antibacterial activity, and O-carboxymethyl chitosan does not undergo a substitution reaction at the - NH2 position, retaining a certain amount of - NH2, making its antibacterial effect relatively strong.
The alkylation modification of chitosan generally occurs on the nitrogen atom of chitosan NH2 and the oxygen atom of - OH, resulting in two different substitution positions of chitosan derivatives, N-alkylated chitosan and O-alkylated chitosan. The modification methods include generating Schiff bases, synthesizing transition metal ions, synthesizing phthalic anhydride, etc. The most common one is the reaction of chitosan with fatty aldehydes to generate Schiff bases, which are then reduced by NaBH4 in acidic solution to obtain N-alkylated chitosan. The reaction process is shown in Figure 4B.
Sulfonation reaction refers to the process in which the - NH2 or - OH of chitosan introduces sulfonic acid groups (- SO3H) or their corresponding salts or sulfonyl halides through chemical reactions with sulfuric acid, sulfur dioxide, or chlorosulfonic acid. The reaction process is shown in Figure 4C. There are negatively charged sulfonic acid groups (RSO3-) on the sulfonated chitosan molecules, which can chelate with the metal ions required for bacterial growth and thus have a certain impact on bacterial growth and development.
The quaternization reaction is currently the longest studied and most widely used method in chitosan chemical modification, mainly including the grafting reaction of quaternary ammonium groups directly introducing small molecules of quaternary ammonium groups onto chitosan amino groups; Chitosan reacts with aldehyde to obtain Schiff base, which is then reduced and reacted with halogenated alkane to obtain chitosan quaternary ammonium salt and grafted quaternary ammonium groups through direct quaternization reaction. The reaction process is shown in Figure 4D. After quaternization reaction modification, chitosan molecules contain protonated - NH2, which gives them good antibacterial properties at low pH values.
2.2 Synergistic antibacterial effect with other substances
Chitosan has good biocompatibility and can synergize with inorganic nanoparticles, essential oils, microbial metabolites, and other substances, greatly enhancing its antibacterial effect.
2.2.1 Inorganic nanoparticles and chitosan synergistically interact in chitosan residues, with one - NH3 at the C2 position and one - OH at the C3 position. The flat bond conformation enables chitosan to strongly chelate metals or metal oxides such as titanium, silver, zinc, copper, and chromium. By utilizing chitosan's chelation of metals, hydrogen bonds in chitosan molecules can be broken, causing the molecular chain to expand and decompose, generating more positive charges and enhancing the antibacterial activity of chitosan. Among them, inorganic nanoparticles have the potential to exert antibacterial effects by adhering to microbial cells, penetrating into cells to destroy intracellular substances, generating reactive oxygen species and free radicals leading to oxidative stress induced cell death, and regulating signal transduction pathways. 1) Metal particle Ag is currently one of the most widely used antibacterial metal materials. Silver ions have empty orbitals and can coordinate with the unused electron pairs of the nitrogen atom of the free NH2 on the chitosan chain to form chitosan silver complexes. Zhao Xixi et al. synthesized silver nanoparticles AgNPs using grape seed extract as a stabilizer and reducing agent, and prepared chitosan nanocomposite coatings by compounding with chitosan. In the experiment of grape fungal contamination, it was found that the total number of grape yeast and mold coated with the composite coating was as low as 2.9 (lg (CFU/g)) during storage. Cu nanoparticles can penetrate the cell wall, causing proteins and other intracellular components to leak, leading to bacterial cell death and exerting antibacterial effects. The use of in-situ reduction method to fix Cu on the surface of chitosan can improve the antibacterial performance of chitosan, making the antibacterial effect of chitosan Cu nanocomposite material stronger than that of original chitosan on Staphylococcus aureus and Candida albicans. 2) Metal oxide nanoparticles can be released within cells, altering cellular structure and function, inducing bacterial oxidative stress, and leading to bacterial death. Metal oxides have the advantages of broad-spectrum, safety, durability, and resistance. Therefore, by combining chitosan and metal oxides to achieve synergistic effects, the antibacterial effect can be further enhanced. Rahman et al. used a one pot method to disperse ZnO nanoparticles in chitosan solution and prepared a novel chitosan ZnO film for packaging beef. It was found that on the 6th day, there was no growth of microorganisms such as Staphylococcus aureus and Escherichia coli, but ZnO nanoparticles were prone to aggregation in the chitosan matrix and the film surface was rough. TiO2 exerts photocatalytic activity by releasing reactive oxygen species, thereby damaging microbial cell walls. Siripatawan et al. added TiO2 to chitosan films and found that when the films were exposed to ultraviolet light, TiO2 underwent photocatalytic reactions, producing reactive oxygen species that increased the permeability of microbial cell membranes, leading to the oxidation of coenzyme A and lipid peroxidation within the cells, ultimately resulting in cell death. By utilizing the excellent antibacterial properties of chitosan and metal ions, and combining their synergistic antibacterial properties organically, the antibacterial performance of the film can be significantly improved. However, the release and toxicity of metal particles are still being questioned. Therefore, further research is needed to develop composite materials that can not only exhibit excellent antibacterial effects on food surfaces but also ensure food safety. 2.2.2 Essential oils or plant extracts synergistically with chitosan. Essential oils are a type of plant secondary metabolite mainly composed of volatile aromatic extracts, among which aldehydes, phenols, and oxygen-containing terpenes are the main antibacterial active substances of essential oils. The main active ingredients of plant extracts include flavonoids, polyphenols, alkaloids, organic acids, etc., which are mostly stable secondary metabolites. The active ingredients in these substances can disrupt cell membrane permeability, leading to abnormal synthesis of intracellular genetic material and proteins, hindering bacterial cell formation, and thus exerting antibacterial effects. It is a highly promising green antibacterial agent. In addition, hydrophilic groups such as flavonoids and saponins in plant extracts can enhance the water solubility of chitosan, making it more antibacterial. Essential oils or plant extracts have potential for synergistic antibacterial activity with chitosan, however, further research is needed in terms of their stability, sustained release, and other aspects. 2.2.3 Collaboration between Microbial Metabolites and Chitosan
Microorganisms can produce various metabolites such as lactobacillus, ε - polylysine (ε - PL), lysozyme, etc. They have strong antibacterial ability, are safe, non-toxic, and easy to cultivate, and are widely used in antibacterial materials. Among them, ε - PL and lactobacillus mainly achieve antibacterial effects by disrupting the cell membrane, causing the loss of intracellular substances, and affecting the interaction between membrane molecules. Lysogenic bacteria mainly cause microbial cell wall hydrolysis and the breakdown of peptidoglycan skeleton substances, leading to microbial lysis and death. The combination of microbial metabolites and chitosan can alleviate the problems of easy degradation and poor antibacterial stability of microbial metabolites, ensuring their antibacterial effect. However, the toxicity of microbial metabolites still needs to be evaluated, and it is necessary to ensure that their usage is within the edible range.
03
Application of Chitosan in New Food Packaging
During the process of food processing, storage, and transportation, microorganisms are prone to cause food spoilage and produce toxic and harmful metabolites, posing a threat to human health. The use of active antibacterial packaging can significantly reduce the level of microbial infection and extend the shelf life of food. Composite preparation of active packaging film by combining polysaccharide, lipid or protein membrane matrix with active antibacterial substance chitosan can endow the film with better mechanical properties, barrier properties and antibacterial activity, thereby delaying food spoilage caused by microorganisms.
3.1 Chitosan polysaccharide composite film
Polysaccharides such as alginate, cellulose, starch, and pullulan polysaccharides are rich in functional groups such as - OH, carbonyl, and - NH2. Chitosan acts on polysaccharides through hydrogen bonding, electrostatic interactions, and other mechanisms, significantly enhancing the antibacterial ability of the overall polysaccharide membrane structure.
Alginate is a natural anionic polymer with good biodegradable, non-toxic, bioadhesive and gel properties. Chitosan and alginate are aquatic polysaccharides, which can be used to prepare antibacterial films through electrostatic interaction and non covalent cross-linking. Cellulose is the most abundant natural polymer in nature, containing easily modifiable - OH, which can serve as a good supporting material for antibacterial agents. Starch is a carbohydrate composed of glucose units connected by glycosidic bonds, and a large number of hydrogen bonds can be formed between polymers. Therefore, it is often used in the development of starch based composite materials. As a microbial derived polysaccharide, pullulan has good solubility and film-forming properties, making it a good thin film substrate. Although polysaccharide composite films are suitable as biodegradable materials for food packaging, they form high molecular weight polymers during polysaccharide composite, making it difficult to evenly coat the film. Secondly, the moisture resistance of polysaccharide composite films is poor, which can affect their mechanical properties in high humidity environments. This also limits the widespread use of polysaccharide composite films. Therefore, improving the performance of food packaging films has become an essential part of current research on polysaccharide film modification.
3.2 Chitosan protein composite membrane
The - NH2 of chitosan carries a positive charge and can interact electrostatically with deprotonated carboxyl groups of proteins, thereby improving the antibacterial storage stability of the composite film. Researchers have conducted in-depth research on the complexation of chitosan with protein biopolymers such as gelatin, soy protein isolate, and zein.
Research has found that gelatin and chitosan can have good miscibility with chitosan through hydrogen bonding and electrostatic interactions. Chitosan and soy protein isolate form a tight structure between molecules through electrostatic interactions, resulting in films with good mechanical and barrier properties. Chitosan has good viscosity under acidic conditions, and its large molecular residues can crosslink with functional groups of zein to form a network structure. Chitosan zein composite materials have good mechanical properties, antibacterial properties, antioxidant properties, and hydrophobicity.
3.3 Chitosan lipid composite membrane
Chitosan can be combined with wax film and vegetable oil film, and the hydrogen bonding between chitosan and beeswax - OH makes the adsorption between the two more compact. Functional chitosan and beeswax have a positive prospect in providing antibacterial barriers for food packaging.
The cationic NH3+of chitosan macromolecules can generate a charge attraction effect with negatively charged oils and fats, thereby preparing composite coatings.
04
epilogue
Chitosan has great potential as an antibacterial material due to its antibacterial activity and non toxicity. By modifying and synergizing with other substances, the antibacterial activity of chitosan antibacterial composite materials can be improved to a certain extent, effectively preventing food spoilage and the growth of pathogenic microorganisms. Chitosan, as a natural antibacterial agent, endows different film-forming substrates with antibacterial activity, effectively extending the shelf life of various foods and other agricultural products, while reducing the use of synthetic plastics and additives, and reducing environmental pollution. Therefore, the development prospects of chitosan in the field of food antibacterial packaging are very broad.
The long-term antibacterial properties, safety, and production costs of food packaging films are still issues facing the industrial promotion of chitosan. Therefore, in future research, the antibacterial stability of chitosan based antibacterial packaging materials under different conditions and the sustained release process of active substances can be further explored to ensure that they can maintain antibacterial activity for a long time; Improving the comprehensive properties of chitosan system, such as mechanical properties, barrier properties, and antibacterial properties, provides possibilities for expanding the application scope of chitosan films; Further research is needed to determine whether food packaging films containing active substances will affect the sensory characteristics of food, such as stimulating odors, during application; The toxicity of chitosan oligosaccharide composite materials, especially composite metal materials, still needs to be closely monitored. The safety evaluation of antimicrobial packaging requires the determination of appropriate methods and relevant standards to ensure the safety of consumers during consumption; Avoiding tedious processes of raw material separation, purification, and membrane formation, improving production efficiency, and reducing production costs.
corresponding author
Lecturer Zhou Hui
The School of Food Science and Engineering at Dalian Ocean University obtained a Bachelor's degree in Chemical Engineering and Technology from Chengdu University of Technology in June 2003, a Master's degree in Chemical Technology from Sichuan University in June 2006, and a PhD in Industrial Catalysis from Dalian Institute of Chemical Physics in January 2017. I worked as a teacher and researcher at the School of Chemistry and Chemical Engineering, Southwest Petroleum University from June 2006 to September 2011. I started working at the School of Food Science and Engineering, Dalian Ocean University in March 2017. Mainly engaged in the separation and purification of marine biological active substances (oligosaccharides, active peptides), preparation of alginate edible films and other research work. In recent years, he has presided over one Young Scientists Fund of the National Natural Science Foundation of China, one project of the Science and Technology Department of Liaoning Province, one project of the Education Department of Liaoning Province, one project initiated by a university doctor, and one open fund of the State Key Laboratory of Catalysis; Apply for 4 national invention patents; More than 20 papers have been published.
Lead author
Liu Mengqi, a master's student
College of Food Science and Engineering, Dalian Ocean University
During my master's degree, I mainly engaged in research on the antibacterial and preservation properties of brown seaweed film, exploring the inhibitory level of films containing antibacterial substances on microorganisms in refrigerated preserved sea bass, providing ideal preservation effects for sea bass fillets, and achieving functional food packaging. Participated in the publication of 3 academic papers, including 3 SCI and EI indexed papers.